What do solar cell panels and the purple bacterium Rhodobacter sphaeroides have in common? Both convert the sun light into other useful forms of energy. And while the physical and chemical processes at the heart of these light-converting systems are very different, the relevant steps of charge excitation and charge and energy transport can be described by the same set of fundamental quantum mechanical equations.
A theoretical physicist by training, I have found myself gravitating towards topics at the nexus of physics, (bio)chemistry and material science. I enjoy working interdisciplinary and communicating with experimentalists and theoreticians alike. In my group, we use and develop accurate electronic structure methods to simulate the electronic structure and dynamics of complex light-harvesting systems, both natural and man-made.
Expertise
Material Science
- Halide
- Density
Chemistry
- Perovskites
- First Principle
- Band Gap
- Electronic State
- Density Functional Theory
- Structure
Organisations
Methods
Density functional theory (DFT) is the method of choice for fast calculations of ground state properties, often with excellent accuracy. Standard approximations, however, lack reliability for some of the most interesting and technologically relevant materials. To compute ground and excited state properties of a wide variety of complex systems, we therefore draw upon state-of-the-art, first-principles DFT and many-body perturbation theory methods. The GW and GW-BSE approximations are particular areas of focus because they allow for the calculation of spectroscopic properties that can readily be compared with results from photoemission and -absorption experiments.
Biological Light-Harvesting Systems
Nature's light harvesting systems, the photosynthetic units of plants, algae and purple bacteria, convert the energy of sunlight to chemical energy in a complicated process of charge excitation, transport, and separation with near 100% quantum efficiency. While the guiding principles of the light harvesting process are well understood today, a full ab initio characterization of charge excitations and transport has not been undertaken. What is more, fundamental questions concerning the role of quantum mechanical coherence as well as electron-phonon coupling in the excitation energy transport to the reaction center in the photosynthetic unit are still unresolved. Our objective is to gain a deep understanding of the quantum mechanical processes at the heart of light harvesting and photosynthesis using first principles DFT and many-body perturbation theory. In addition, our work is motivated by a desire to understand the design principles that enable near-perfect quantum efficiencies under the diverse and sometimes deleterious environmental conditions that photosynthetic organisms are presented with in nature. We hope to contribute to an understanding of how these design principles can be adapted to man-made systems.
Halide Perovskites
Perovskites are a rich family of materials that are being studied intensely due to a wealth of properties including ferroelectricity, ferromagnetism, superconductivity and many more. Only recently, hybrid organic-inorganic halide perovskites have been (re)discovered as highly efficient photovoltaic absorber materials and solar cells based on such perovskites have exceeded power conversion efficiencies of 22%. Their complex phase diagrams, intricate structure, and multi-scale phenomenology, however, pose challenges to theory and experiment alike. In close collaboration with experimentalists, we are working on computing accurate spectroscopic properties to better understand and tune the electronic structure of existing halide perovskites and predict new, environmentally benign materials.
Transition- and noble metal clusters and nanoparticles
Transition- and noble metal nanoparticles are potent catalysts of a variety of technologically relevant reactions. Interestingly, turnover rates of such catalysts can oftentimes be boosted by alloying with another metal. Nanoalloys from Ni and Pd have, for example, shown significantly higher turnover rates in hydrogenation reactions than either of the pure metals. At the same time, clusters and nanoparticles alloyed or doped with transition metals are prime examples for systems that standard DFT cannot handle due to self-interaction errors and a lack of non-locality in its approximations. As such, these systems can serve as models for understanding why and when DFT approximations fail and help to develop better methods for realistic systems.
Publications
2024
2023
Research profiles
Courses academic year 2024/2025
Courses in the current academic year are added at the moment they are finalised in the Osiris system. Therefore it is possible that the list is not yet complete for the whole academic year.
- 193599010 - Internship
- 193599039 - Master Thesis: Physics Aspects
- 193599089 - Master Thesis: General Aspects
- 201700185 - Internship
- 201800344 - Master's Assignment: Physics Aspects
- 201800345 - Master's Assignment: General Aspects
- 202000716 - Bachelor Assignment
- 202001433 - Bachelor’s Assignment AM-AP
- 202100210 - Electronic Structure Theory
- 202200046 - Light and Matter
- 202200093 - Quantum Mechanics 2
- 202400415 - Proc. and Mat. for Sust. Energy Convers.
- 202400659 - Combined Final Project EE / AP
Courses academic year 2023/2024
- 193599010 - Internship
- 193599039 - Master Thesis: Physics Aspects
- 193599089 - Master Thesis: General Aspects
- 201700185 - Internship
- 201800344 - Master's Assignment: Physics Aspects
- 201800345 - Master's Assignment: General Aspects
- 202000716 - Bachelor Assignment
- 202001432 - Bachelor’s Assignment AM-AP
- 202001433 - Bachelor’s Assignment AM-AP
- 202100210 - Electronic Structure Theory
- 202200046 - Light and Matter
- 202200093 - Quantum Mechanics 2
- 202200258 - Double Master Thesis: NT-AP
Address
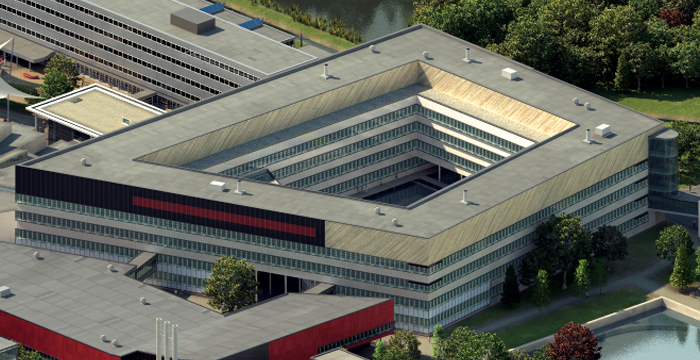
University of Twente
Carré (building no. 15)
Hallenweg 23
7522 NH Enschede
Netherlands
University of Twente
Carré
P.O. Box 217
7500 AE Enschede
Netherlands